No Temperature Controls Necessary For Small Molecules? Are You Sure?
Ensuring Quality for Small Molecule Therapy Supply Chains in a Growing Market Managing the...
read Details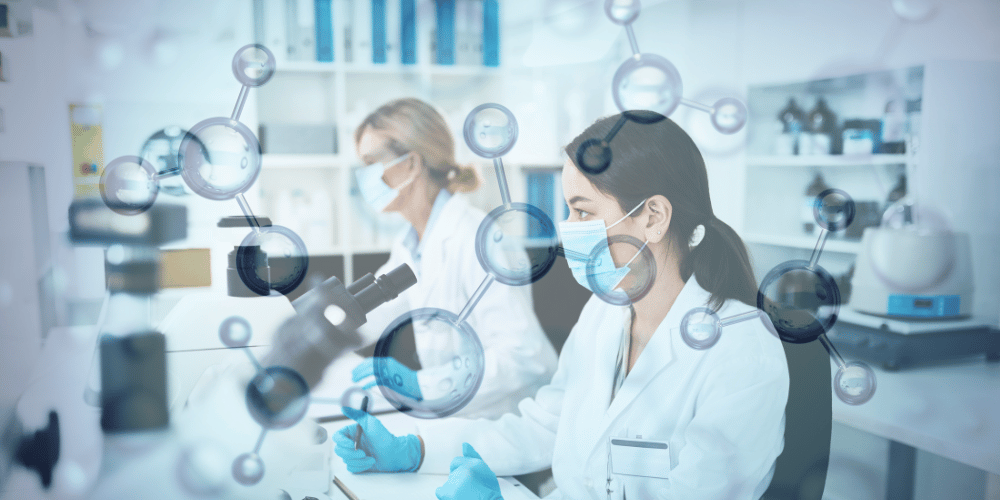
by Carson Dickey, Senior Consulting Engineer and Sarah Williamson, Consulting Engineer
mRNA therapies are an exciting and rapidly growing class that have the potential to combat many prevalent diseases and disorders. However, the storage and shipping requirements of mRNA therapies tend to require the use of expensive and complex deep frozen cold chains. As of March 2022, there were 823 mRNA therapies in development [1] (preclinical to pre-registration) and as of July 2022, there were only thirteen (13) approved mRNA therapies in the US [2]. The use of mRNA therapies is new to the pharmaceutical industry, and is expected to grow expeditiously over the next decade.
In late 2022, I had the opportunity to attend the 10th International mRNA Health Conference. The event was full of engaging topics but there was a distinct lack of discussion regarding the temperature stability of mRNA therapies and how that relates to commercialization and the operation of a cold chain. Stability and the expected shipping and storage conditions for a therapy are extremely important and should be considered early on in the drug development process.
Most current mRNA therapies operate in frozen (-20 °C) or deep frozen (-80 °C) cold chains. While this is feasible, there are significant cost savings and environmental impacts for using a warmer, refrigerated cold chain as opposed to a deep-frozen cold chain.
Storing a therapy in a deep-frozen freezer at -80 °C can use up to four (4) times more energy than storing at 5 °C. Furthermore, the cost for a deep frozen freezer can be three (3) times more expensive vs a storage unit that operates at refrigerated temperatures. Shipping deep frozen therapies require the use of dry ice, which can increase shipping costs due to the additional package weight that dry ice adds as well as the cost to purchase the dry ice itself. Additional weight during shipping can also increase emissions, further adding to the environmental cost of storing and shipping in a deep-frozen supply chain.
There are obvious benefits to moving away from deep frozen supply chains, and efforts are being made to improve temperature stability of mRNA therapies, such as the use of excipients (e.g., stabilizers and/or the inclusion of preservatives), formulation milieu (e.g., pH and tonicifying agents), and manufacturing processes (e.g., liquid or lyophilized dosage forms) [3]. While temperature stability has improved these methods, shipping at refrigerated temperatures may cause mRNA products to be more vulnerable to distribution hazards such as shock and vibration.
Studies have shown that vibration and shock can greatly reduce efficacy of COVID mRNA vaccines at temperatures above freezing. One study on the Pfizer COVID-19 vaccine showed that vibration and shock can cause up to a 49.8% and a 15.0% reduction in efficacy, respectively, due to the release of mRNA from the lipid nanoparticles [4]. The study also showed that vibration causes aggregation which reduces the efficacy of the vaccine. Another study on mRNA encapsulated in lipid nanoparticles (LNPs), strong vibration caused “a clear collapse of the nanoparticle structure” due to aggregation [5].
As more mRNA therapies are developed, the stability and cold chain requirements of the therapy must be considered to avoid complications as these therapies are commercialized. The impact of shock, vibration, and temperature must be explored during formulation development to avoid the use of expensive deep frozen cold chains and to ensure that the therapy is not adversely impacted by distribution hazards such as shock and vibration.
No matter your modality or therapeutic use, Modality Solutions has the expertise to optimize the cold chain and guide your therapy through the regulatory framework from early development through approval. Our transport simulation testing technology and regulatory experience span the full range of therapeutic modalities and their diverse cold chain requirements. Don’t hesitate to reach out to our team to discuss your cold chain needs.
[2] Zhu, Y., Zhu, L., Wang, X. et al. RNA-based therapeutics: an overview and prospectus. Cell Death Dis 13, 644 (2022). https://doi.org/10.1038/s41419-022-05075-2
[3] Daan J.A. Crommelin, Thomas J. Anchordoquy, David B. Volkin, Wim Jiskoot, Enrico Mastrobattista, Addressing the Cold Reality of mRNA Vaccine Stability, Journal of Pharmaceutical Sciences, Volume 110, Issue 3, 2021, Pages 997-1001, ISSN 0022-3549, https://doi.org/10.1016/j.xphs.2020.12.006.
[4] Kudsiova L, Lansley A, Scutt G, Allen M, Bowler L, Williams S, Lippett S, Stafford S, Tarzi M, Cross M, Okorie M. Stability testing of the Pfizer-BioNTech BNT162b2 COVID-19 vaccine: a translational study in UK vaccination centres. BMJ Open Sci. 2021 Sep 12;5(1):e100203. doi: 10.1136/bmjos-2021-100203. PMID: 35047705; PMCID: PMC8647588.
[5] Kamiya M, Matsumoto M, Yamashita K, Izumi T, Kawaguchi M, Mizukami S, Tsurumaru M, Mukai H, Kawakami S. Stability Study of mRNA-Lipid Nanoparticles Exposed to Various Conditions Based on the Evaluation between Physicochemical Properties and Their Relation with Protein Expression Ability. Pharmaceutics. 2022; 14(11):2357. https://doi.org/10.3390/pharmaceutics14112357
Ensuring Quality for Small Molecule Therapy Supply Chains in a Growing Market Managing the...
read DetailsEnsuring the safe, effective delivery of small molecule drugs is more challenging than ever....
read DetailsAt a Glance: Cold Chain Engineering: Modality Solutions focuses on enhancing drug delivery through...
read Details